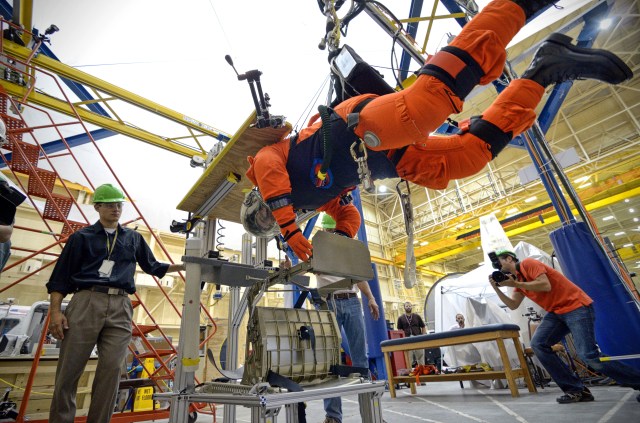
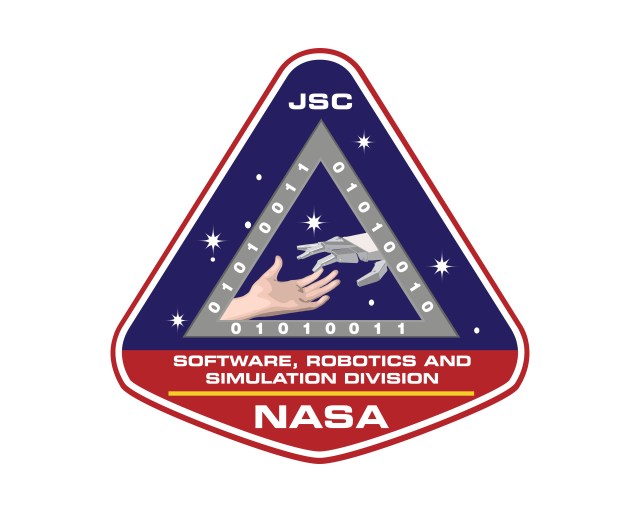
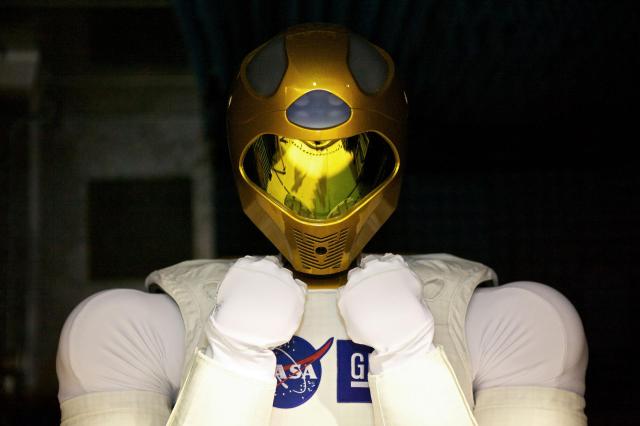
Software, Robotics, and Simulation Division
Established in 1990, the Software, Robotics, and Simulation Division (ER) is responsible for the design, development, testing, and operations of intelligent systems, robotic systems, and real-time simulation systems that facilitate the human exploration and development of space. The division participates in both programmatic functions as well as targeted research and development in support of Johnson Space Center programs. The division is organized into several branches.
ER MISSION STATEMENT
The mission of the Software, Robotics, and Simulation Division is to enable the human exploration of space, and contribute to the achievement of national goals, through the development, adaptation, and infusion of system technologies for automation, robotics, flight software, simulation, graphics, and exercise equipment into the engineering and operations of current and future spacecraft, and surface systems for the purposes of improving their capability, operability, reliability, safety, and life cycle costs.
ER VISION STATEMENT
We will enable the expansion of the frontiers of space, and the achievement of national goals, through the development and application of system technology for automation, robotics, flight software, simulation, graphics, and exercise equipment.
ER CHARTER
The Software, Robotics, and Simulation Division is responsible for defining requirements, analyzing, designing, assembling, integrating, testing, evaluating, verifying, operationally supporting, and managing current and advanced software and hardware systems, in the areas of automation, robotics, flight software, simulation, graphics, and exercise equipment for human surface and space flight operations.
ER Branches
Explore NASA Johnson Space Center's ER branches.
NASA JSC ER YouTube
Watch now about NASA JSC ER YouTube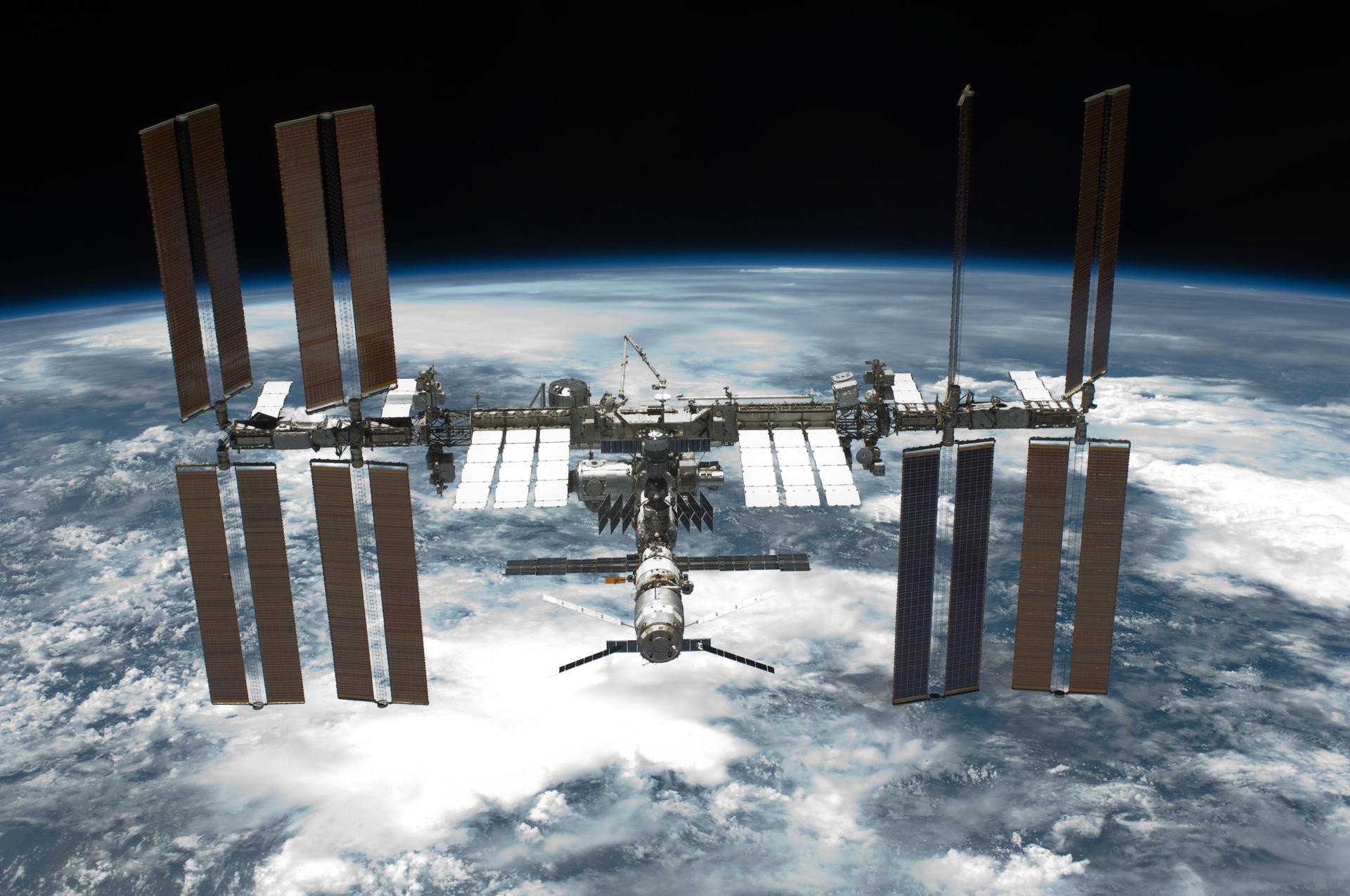